Différences entre les versions de « Abstract pool for the NCI workshop »
Ligne 828 : | Ligne 828 : | ||
[http://wiki.lct.jussieu.fr/workshop/index.php/Abstract_pool_for_the_NCI_workshop ↑ top of this page] | [http://wiki.lct.jussieu.fr/workshop/index.php/Abstract_pool_for_the_NCI_workshop ↑ top of this page] | ||
− | == | + | ==Gabriele Saleh== |
− | '' | + | ''Department of Chemistry, Università degli Studi di Milano, via C.Golgi 19, 20159 Milano Italy'' |
− | <big>''' | + | <big>'''exploring non-covalent interactions in molecular crystals through the analysis of their experimental electron density distribution'''</big> |
+ | |||
+ | The so-called ‘NCI descriptor’ introduced by Johnson et al. [1], besides allowing an easy-to-catch pictorial visualization of Non-Covalent Interactions (NCI), presents the big advantage of being based on electron density (ED) distribution, allowing therefore to investigate NCI theoretically and experimentally on the same ground. Indeed, ED is an observable and measurable quantity (from X-ray diffraction experiments) which, at the same time, can be straightforwardly obtained from the wavefunction. | ||
+ | Quite recently, we have applied the 'NCI descriptor' for the first time to experimentally derived ED [2]. We have also compared the experimental results with the one obtained from fully periodical DFT calculation, to assess the reliability of experimentally derived NCI picture. Moreover, we have contrasted the picture obtained from 'NCI descriptor' with the one obtained from Quantum Theory of Atoms in Molecules [3] to show how the two approaches may fruitfully complement each other in providing additional insight into the nature of a given NCI. In a separated work [4] we carried out a systematic comparison between the NCI picture obtained from promolecular ED (i.e. the ED obtained by superposing the atomic densities) and the one from experimentally derived ED, to explore to what extent the promolecular ED can be used to investigate NCI, as proposed by Contreras et al. [5] . | ||
+ | The results relative to the application of NCI descriptor to experimental, theoretical and promolecular ED of several system will be presented. In addition, preliminary results related to a variation of the classical 'NCI descriptor', exploiting the energy density, will be shown. | ||
+ | |||
+ | References: | ||
+ | [1]E. R. Johnson, S. Keinan, P. Mori-Sanchez, J. Contreras-Garcia, A. J.Cohen, W. Yang, J. Am. Chem. Soc. 2010, 132, 6498 – 6506 | ||
+ | [2]G. Saleh, C. Gatti, L. Lo Presti, J. Contreras-Garcia, Chem. Eur. J. 2012, 18, 15523–15536 | ||
+ | [3]R. F. W. Bader, Atoms In Molecules: A Quantum Theory, Clarendon Press, Oxford, 1990 | ||
+ | [4]G. Saleh, C. Gatti, L. LoPresti, Comput. Theor. Chem. 2012,998,148–163 | ||
+ | [5]J. Contreras-Garcia, E.R. Johnson,S. Keinan, R.Chaudret , J-P. Piquemal, D.N. Beratan, W. Yang, J. Chemical Theory and Computation, 2011, 7, 625-632 | ||
− | |||
[http://wiki.lct.jussieu.fr/workshop/index.php/Abstract_pool_for_the_NCI_workshop ↑ top of this page] | [http://wiki.lct.jussieu.fr/workshop/index.php/Abstract_pool_for_the_NCI_workshop ↑ top of this page] | ||
− | |||
==Your name== | ==Your name== |
Version du 26 avril 2013 à 21:33
<<< Topological Approaches to Intermolecular Interactions workshop main page
You can add your abstract below:
- first : log in;
- click on "edit", on the righthand corner above the the line below "Your name"
- copy the example given (also available >>> here) and paste it at the end of this page
- fill in the fields with your data
- >>> How to insert a picture in your abstract
- Don't forget to send us an email with the type of contribution you would like to make
Plenary Speakers
Paul Popelier
To be submitted
Frank De Proft
Eenheid Algemene Chemie (ALGC), Vrije Universiteit Brussel (VUB), Pleinlaan 2, B-1050 Brussels, Belgium
Combined use of DFT based Reactivity Indices and the Non-covalent Index in the Study of Intermolecular Interactions
Conceptual Density Functional Theory (sometimes also called DFT based reactivity theory or Chemical DFT) has proven to be an ideal framework for the introduction of chemical reactivity descriptors [1]. These indices are defined as response functions of the energy E of the system with respect to either the number of electrons N, the external potential v(r) or both. These definitions have afforded their non-empirical calculation and applications in many fields of chemistry have been performed, often combined with principles such as the electronegativity equalization principle of Sanderson and Pearson’s hard and soft acids and bases (HSAB) and maximum hardness principles. In this talk, we focus on recent studies in which a combined use of these reactivity indices and the Non-Covalent Interaction (NCI) Index [2] was carried out in order to scrutinize intermolecular interactions. In a first part, we focus on halogen bonding. Halogen bonds between the trifluoromethyl halides CF3Cl, CF3Br and CF3I, and dimethyl ether, dimethyl sulfide, trimethylamine and trimethyl phosphine were investigated using the HSAB concept with conceptual DFT reactivity indices, the Ziegler-Rauk type energy decomposition analysis [3], the Natural Orbital for Chemical Valence framework (NOCV) [4], and the NCI index. It is found that the relative importance of electrostatic and orbital (charge transfer) interactions in these halogen bonded complexes varies as a function of both the donor and acceptor molecules [5]. Hard and soft interactions were distinguished and characterized by atomic charges, electrophilicity and local softness indices. Dual descriptor plots indicate an orbital σ-hole on the halogen similar to the electrostatic σ-hole manifested in the molecular electrostatic potential. The characteristic signal found in the reduced density gradient versus electron density diagram corresponds to the non-covalent interaction between contact atoms in the NCI plots, which is the manifestation of halogen bonding within the NCI theory. The unexpected C–X bond strengthening observed in several cases was rationalized within the MO framework.
In a second part of the talk, we present recent results on the use of the above mentioned quantities in the study of metal-metal interactions [6].
References
[1] (a) R. G. Parr and W. Yang, Density Functional Theory of Atoms and Molecules, Oxford University Press, New York, 1989. (b) R. G. Parr and W. Yang, Ann. Rev. Phys. Chem. 46, 701 (1995). (c) H. Chermette, J. Comput. Chem. 20, 129 (1999). (d) P. Geerlings, F. De Proft and W. Langenaeker, Chem. Rev. 103, 1793 (2003). (e) P. W. Ayers, J. S. M. Anderson and L. J. Bartolotti, Int. J. Quant. Chem. 101, 520 (2005).
[2] (a) E. R. Johnson, S. Keinan, P. Mori-Sanchez, J. Contreras-Garcia, A. J. Cohen and W. T. Yang, Journal of the American Chemical Society 132, 6498 (2010). (b) J. Contreras-Garcia, E. R. Johnson, S. Keinan, R. Chaudret, J. P. Piquemal, D. N. Beratan and W. T. Yang, J. Chem. Theor. Comput. 7, 625 (2011).
[3] (a) F. M. Bickelhaupt and E. J. Baerends, Reviews in Computational Chemistry, 15, 1 (2000); (b) T. Ziegler and A. Rauk, Theoretica Chimica Acta 46, 1 (1977).
[4] M. P. Mitoraj, A. Michalak and T. Ziegler, J. Chem. Theor. Comput. 5, 962 (2009).
[5] B. Pinter, N. Nagels, W. A. Herrebout and F. De Proft, Chem. Eur. J. 19, 518 (2013).
[6] B. Pinter, L. Broeckaert, J. Turek, A. Růžička and F. De Proft, in preparation.
Weitao Yang
To be submitted
Manuel Yáñez
Departamento de Química. Facultad de Ciencias. Módulo 13. Universidad Autónoma de Madrid. Campus de Excelencia UAM-CSIC. Cantoblanco. 28049-Madrid. Spain.
Playing around with Beryllium Bonds
Beryllium bonds share many characteristics with conventional hydrogen bonds (HBs) [1], and as in HBs the proton donor may become significant distorter, the formation of Be bonds are also accompanied by a dramatic distortion of the beryllium derivative. This deformation plays a crucial role as far as the relative stability trends are concerned since it amounts to about a 30% of the total interaction energy, but more importantly significantly enhances the electron acceptor capacity of Be containing Lewis acid [2]. When these two closed-shell kinds of interactions are present in the same system, like in the complexes between BeX2 derivatives and imidazole dimer, clear cooperativity effects are observed, which result in a mutual reinforcement of both non-covalent interactions. This permits to use the Be bonds to modulate or tune the strength of inter- and intra-molecular HBs [3]. Indeed, for more complex clusters, as those involving water trimers, both cooperative and anti-cooperative effects are found [4]. More importantly Be bonds lead to a significant change in the intrinsic properties of the Lewis base participating in the bond, in particular on its intrinsic acidity which is dramatically enhanced [5]. The possibility of building up ditopic systems, in which the acid site is a BeX group opens interesting possibilities to design new polymeric materials.
[1] M. Yáñez., P. Sanz, O. Mó, I. Alkorta and J. Elguero, J. Chem Theor. Comput. 2009, 5, 2763-2771.
[2] A. Martín-Sómer, A. M. Lamsabhi, O. Mó and M. Yáñez, Comput. Theor. Chem. 2012, 998, 74-79.
[3] O. Mó, M. Yáñez, I. Alkorta and J. Elguero, J. Chem. Theory Comput. 2012, 8, 2293-2300.
[4] L. Albrecht, R. J. Boyd, O. Mo and M. Yanez, Phys. Chem. Chem. Phys. 2012, 14, 14540-14547.
[5] O. Mó, M. Yáñez, I. Alkorta, J. Elguero, J. Mol. Mod. 2013. dx.doi.org/10.1007/s00894-012-1682-y
Contributions
Odile Eisenstein
To be sumbitted
Mauro Causà
Deparment of Chemical and Materials Engineering, Universita' di Napoli "Federico II"
Maximum Probability Domains in molecular crystals and surfaces
The Maximum Probability Domain (MPD) analysis due to Andreas Savin is applyed to molecular crystals and their surfaces. The correlation effects are included using Quantum Monte Carlo techniques. The MPD correlated method will be applied to rare gas solids, organic materials applied in photo-electronics, and to crystal phases relevant in heterogeneous atmospheric chemistry.
Piotr Kasprzycki, Piotr Ciąćka, Piotr Fita, Czesław Radzewicz
Institute of Experimental Physics, Faculty of Physics, University of Warsaw, ul. Hoza 69, 00-681 Warsaw, Poland
The correlation between the proton transfer time and electron density in the inner cavity of porphycenes
Numerical solutions of the Schrödinger equation can nowadays be obtained for reasonably large organic and organometallic molecules with useful accuracy using approximate ab initio or DFT methods. These approximate solutions are also available for porphycene which is an important chromophore [1]. Porphycene and its derivatives show useful properties for material sciences and are also considered excellent photosensitive media for applications in photodynamical therapy of cancer [2]. Several papers have discussed the energy structure and dynamic aspects of hydrogen transfer in this systems [4, 5]. We report on the results of NCI analysis - theoretical tool based on Bader approach [6] - performed for symmetrically and asymmetrically substituted porphycene derivatives. We have carried out DFT calculations at the level B3LYP/6-311(d,p) of theory. These calculations were followed by NCI analysis. Calculations showed a correlation between the value of electron density gradient localized between nitrogen and hydrogen atoms in the inner cavity of a molecule and the time of the proton transfer in the examined systems. Proton transfer times were obtained in a femtosecond transient absorption anisotropy experiment, which is a polarisation-sensitive variant of an ultrafast pump-probe technique. Double hydrogen transfer leads to the change of transition dipole moment directions - transition moment of chemically identical tautomerization product forms an angle of α = (72±2)◦ with that of initially excited form. This change is manifested as variation in transmitted light intensity measured in two orthogonal polarizations. Under appropriate conditions, rise/decay times of anisotropy reflect the kinetics of double hydrogen transfer. We show that quantum theory of atoms in molecules (QTAIM) based on Bader approach is useful not only for detection of covalent bond but also for hydrogen bonds and weak van der Waals interaction. NCI analysis provides the possibility to compare strength of hydrogen bonds in porphycene derivatives, which allows one to predict the proton transfer time for new molecules. Additionally, these approach have helped us to design structure of a derivative with the proton transfer time of the order of tens of picoseconds. Furthermore, substitution in 2,7 and in 10,19 position of porphycene can induce differentiation of hydrogen bonds. The effect is especially evident in the case of 10,19-di-methylporphycene (2MPc) and 2,7-di-t-buthylporphycene (DTBP). This behavior may be the result of asymmetric distribution of electron density in the center of the molecule. This fact can be significant for understanding the mechanism of double hydrogen transfer between inner nitrogen atoms.
References
[1] D. Sanchez-Garcia, J. L. Sessler, Chem. Soc. Rev., 37, 215, (2008).
[2] J. C. Stockert, M. Canete, A. Juarranz, A. Villanueva, R. W. Horobin, J. I. Borrell, J. Teixido, S. Nonell, Curr. Med. Chem., 14, 997, (2007) .
[4] Ł. Walewski, J. Waluk, B. Lesyng, J. Phys. Chem. A., 114, 2313–2318, (2010).
[5] M. Gil, J. Waluk J. Am. Chem. Soc. 5, 129, (2007).
[6] E. R. Johnson, S. Keinan, , J. Contreras-García, R. Chaudret, J-P Piquemal, D. Beratant, W. Yang, J. Chem. Theo. and Comp., 25, (2011).
Slawomir J. Grabowski
Faculty of Chemistry, University of the Basque Country UPV/EHU, and Donostia International Physics Center (DIPC),P.K. 1072, 20080 Donostia, Spain IKERBASQUE, Basque Foundation for Science, 48011 Bilbao, Spain
Non-covalent interactions: characteristics and mechanisms of formation - the topological approach
Numerous non-covalent interactions are characterized by the electron charge transfer from the Lewis base unit to the Lewis acid [1]. This is connected with the other processes reflected by the change of geometrical, energetic and topological parameters. For example, different characteristics of the hydrogen bond and various criteria of the existence of this interaction were discussed in the literature [2]. One can mention the topological criteria of Koch and Popelier [3,4]. On the other hand, the hydrogen bond mechanism was discussed in terms of NBO method [5]. Very recently it was found that the hydrogen bond, the halogen bond and other non-covalent interactions are steered by the same processes [6]. This is reflected by the same changes of parameters. For example, the A-H...B hydrogen bond formation is connected with the increase of the positive charge of H-atom and the decrease of its volume. The same changes are observed for X-halogen atom in the A-X...B halogen bond. Various similarities and differences between numerous non-covalent interactions may be discussed.
References
[1] Lipkowski, P.; Grabowski, S. J.; Leszczynski, J. J. Phys. Chem. A 2006, 110, 10296–10302.
[2] Grabowski, S.J. Chem.Rev. 2011, 11, 2597-2625.
[3] Koch, U.; Popelier, P.L.A. J.Phys.Chem. 1995, 99, 9747-9754.
[4] Popelier, P. Atoms in Molecules. An Introduction, Prentice Hall, Pearson Education Limited 2000.
[5] Alabugin, I.V.; Manoharan, M.; Peabody, S.; Weinhold, F. J.Am.Chem.Soc. 2003, 125, 5973-5987.
[6] Grabowski, S.J. Phys.Chem.Chem.Phys. accepted
Vincent Tognetti and Laurent Joubert
Normandy Univ., COBRA UMR 6014 & FR 3038, Mont Saint Aignan, 76821 cedex, France
QTAIM/DFT descriptors for the description of “weak” interactions
We present here part of our recent work, which aims to design new molecular and atomic descriptors by building a bridge between Density Functional Theory (DFT) and Bader’s Atomsin- molecules (AIM) theory,1 two frameworks that share the same primary ingredient: the electron density. A local point of view will first be presented, dealing with the so-called bond critical points (BCPs). New BCP properties will be investigated,2,3,4,5 based on reduced density gradients and on the variations rates of kinetic, exchange and correlation density energies. Interestingly, these quantities reveal particularly suited to the description of weak interactions, like hydrogen or agostic bonds. Then, we will discuss,6 using Pendás’ Interacting Quantum Atoms scheme,7 to what extent the existence or the absence of such BCPs can be rationalized in terms of integrated interatomic energies, enlightening the fundamental role of exchange channels.8 We will consequently present a fast and accurate protocol for the evaluation of the exchange interaction between two atoms,9 making another promising step in the pathway linking DFT and AIM.
References
[1] R. F. W. Bader, Atoms in Molecules: A Quantum Theory, Oxford University Press (1994).
[1] V. Tognetti, L. Joubert, P. Cortona and C. Adamo, J. Phys. Chem. A 113 12322-12327 (2009).
[3] V. Tognetti, L. Joubert and C. Adamo, J. Chem. Phys. 132 211101 (2010).
[4] V. Tognetti and L. Joubert, J. Phys. Chem. A 115 5505-5515 (2011).
[5] V. Tognetti, L. Joubert, R. Raucoules, T. De Bruin and C. Adamo, J. Phys. Chem. A 116 5472-5479 (2012).
[6] V. Tognetti and L. Joubert, J. Chem. Phys. 138 024102 (2013).
[7] M. A Blanco, A. Martín Pendás and E. Francisco, J. Chem. Theory Comput. 1 1096-1109 (2005).
[8] A. Martín Pendás, E. Francisco, M. A. Blanco and C. Gatti, Chem. Eur. J. 13 9362-9371 (2007).
[9] V. Tognetti and L. Joubert, in preparation.
Agnieszka Roztoczyńska, Justyna Kozłowska, Paweł Lipkowski, Wojciech Bartkowiak
Theoretical Chemistry Group, Institute of Physical and Theoretical Chemistry, Wrocław University of Technology, Wybrzeże Wyspiańskiego 27, 53-370, Wrocław, Poland
Influence of spatial confinement on the cooperativity in the hydrogen-bonded clusters
Cooperativity is a well-known phenomenon and a steering factor of many chemical and physical properties of molecular matter [1-5]. In the present contribution the influence of chemical compression on the hydrogen bond cooperativity has been investigated. For that purpose the cooperativity effects in the isolated and confined (HF)n and (HCN)n clusters were considered. Two models of spatial confinement have been applied in our study. The first one is the cylindrical model harmonic potential, which certainly reflects the general aspects of the confinement effect. The second, less simplified model based on the supermolecular approach: molecular cages within which one can distinguish helium and carbon nanotubes. In order to get an insight into the nature of the cooperative effects in confined spaces a detailed analysis of the energetics and the topological features of electron density according to the concept of the “Quantum Theory of Atoms in Molecules” (QTAIM) proposed by Bader [6,7] have been performed. The properties in question have been analyzed using the ONIOM (M06-2X/6-311++G(2df,2pd):M06-2X/6-31G(d)) method. Moreover, in the case of the harmonic potential calculations, the second-order Møller-Plesset perturbation theory (MP2) has been applied as well. As a part of the study, the comparison of the data obtained within different models of orbital compression have been performed in order to establish the correspondence between the chemical environment and its approximate representations.
[1] A. Korpfen, J. Phys. Chem. 100, 13474 (1996).
[2] T. Kar, S. Scheiner, J. Phys. Chem. A 108, 91619168 (2004).
[3] M. Ziółkowski, S. J. Grabowski, J. Leszczyński, J. Phys. Chem. A 110, 65146521 (2006).
[4] H.J. Song, H.M. Xiao, H.S. Dong, J. Chem. Phys. 125, 074308 (2006).
[5] S. J. Grabowski, Theor. Chem. Acc. 132, 1347 (2013).
[6] R. F. W. Bader, Atoms In Molecules, A Quantum Theory; Oxford University Press: Oxford, U.K., 1990.
[7] R. F. W. Bader, Chem. Rev. 91, 893 (1991).
Paweł Lipkowski, Justyna Kozłowska, Agnieszka Roztoczyńska, Wojciech Bartkowiak
Theoretical Chemistry Group, Institute of Physical and Theoretical Chemistry, Wrocław University of Technology, Wybrzeże Wyspiańskiego 27, 50-370 Wrocław, Poland
Behavior of model hydrogen bonded complexes under the influence of external pressure
Despite of the numerous theoretical and experimental investigations concerning the hydrogen bond phenomenon as well as to the confinement effect there is still a small number of reports on the behavior of the H-bonded systems in the presence of external pressure [1-4]. In order to render the influence of pressure one can apply a model confining potentials which allows to describe the pure valence repulsion contribution. In the present study we have investigated the influence of external pressure, represented by the cylindrical harmonic potential V_conf=1/2(x^2+y^2)ω^2, on the selected linear hydrogen bonded complexes. The model dimeric systems: HF…HF, HCN…HCN, HCN…HCCH have been chosen as a case study. All calculations have been performed employing B3LYP, M06-2X and MP2 methods together with the 6-311++G(2df,2pd) basis set. Moreover, the varied external pressure has been controlled by the oscillator strength (ω=0.0-0.8). The studied complexes have been oriented along the z-axis of the Cartesian coordinate system and fully optimized in the presence of the two-dimensional harmonic oscillator potential. A detailed analysis of changes in topological parameters of hydrogen bonds resulting from the orbital compression has been performed according to the concept of the “Quantum Theory of Atoms in Molecules” (QTAIM) proposed by Bader [5,6]. Furthermore, an energetic analysis has shown a different manner of the interaction energy in the studied H-bonded complexes.
[1] W. Wang, D. Wang, Y. Zhang, B. Ji, A. Tion, J. Chem. Phys. 134 (2011) 054317.
[2] M.C. Gordillo, J. Marti, Chem. Phys. Lett. 329 (2000) 341.
[3] M. Jabłoński, M. Solà, J. Phys. Chem. A 114 (2010) 10253.
[4] G. Miño, R. Contreras, Chem. Phys. Lett. 486 (2010) 119.
[5] R. F. W. Bader, Atoms In Molecules, A Quantum Theory; Oxford University Press: Oxford, U.K., 1990.
[6] R.F. Bader, Chem. Rev. 91 (1991) 893.
Daniela Josa
Centro Singular de Investigación en Química Biolóxica e Materiais Moleculares (CIQUS), Universidade de Santiago de Compostela, Rúa Jenaro de la Fuente, s/n, Santiago de Compostela, 15782, Spain
A DFT-D study of stacking interactions between buckybowls and fullerenes
The first strong evidence of π···π interactions between corannulene and C60 was published in 2007, when Sygula and co-workers synthesized the molecular tweezers made up two units of corannulene that can trap one fullerene (Figure 1) [1]. Since then, the interest by concave-convex π···π interactions has been revived. The modification of the tweezers by a different functionalization of buckybowls, which constitute the main part of tweezers, could improve their efficiency and selectivity. Therefore, the aim of this work is to carry out a detailed study of the effects that can enhance the stacking interactions between buckybowls and fullerenes to achieve our ultimate goal that is predict how to modify the molecular tweezers to improve their efficiency and selectivity. All complexes studied were optimized at the B97-D/TZVP level using resolution of identity approximation (RI) implemented in TURBOMOLE 5.10 program suite [2]. Counterpoise corrections were applied to all reported interaction energies [3].
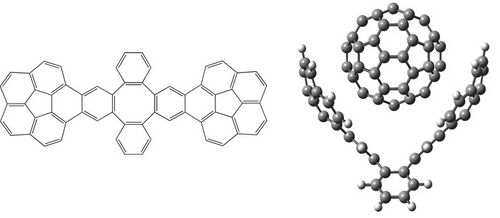
References
[1] A. Sygula, F.R. Fronczek, R. Sygula, P. W. Rabideau, M. M. Olmstead. J. Am. Chem. Soc. 2007, 129, 3842-3843.
[2] R. Ahlrichs, M. Bär, M. Häser, H. Horn, C. Kölmel. Chem. Phys. Lett. 1989, 162, 165-169.
[3] S.F. Boys, F. Bernardi. Mol Phys. 1970, 19, 553-566.
A. Martín Pendás
Universidad de Oviedo. Spain
Decay rate of delocalization indices: towards a real space image of the insulating or metallic character of a material
Real space theories of the chemical bond have come of age, and are now grouped together under the umbrella name of Quantum Chemical Topology (QCT) [1], as originally proposed by Paul Popelier. Most of what is known in chemical bonding has been successfully reformulated using QCT techniques, that provide a wealth of quantities which are invariant under general orbital transformations. However, there is still no clear indication of what type of real space quantities, if any, might be used to discriminate between metals and insulators, in spite of several initial proposals, like those of Gatti and Silvi, or Contreras et. al. Recently, Baranov and Kohout have proposed that localization or delocalization indices might hold the clue [4]. However, their unrestricted Kohn-Sham approach is not devoid of possible criticisms. Here we address the problem from a molecular point of view, examining the analytic behavior of localization and delocalization indices in analytically solvable models and pointing towards the rate of decay of these quantities as a possible measure of long-range (metallic-like) or short-range (insulating-like) electron delocalization in materials [5].
[1] Paul L. A. Popelier, Struct. Bond. 2005, 115, 1.
[2] B. Silvi, C. Gatti, J. Phys. Chem. A, 2000, 104.
[3] J. Contreras-García, A. Martín Pendás, J. M. Recio, B. Silvi , J. Chem. Theory Comput. , 2009, 5, 164.
[4] A. Baranov, M. Kohout, Acta Cryst. A 2011, 67, C115.
[5] A. Martín Pendás, in preparation.
Olga Syzgantseva, Vincent Tognetti, Laurent Joubert
UMR CNRS 6014 & FR 3038, Université de Rouen et INSA de Rouen
Analysis of halogen bonding using topological indexes and IQA energy decomposition scheme
Halogen bonding is a directional interaction occurring between a halogen and an electronegative atom of a Lewis base. It is namely responsible for ligand binding and molecular folding in biological systems and is of importance in crystal engineering [1,2]. While predominantly electrostatic character of this interaction seems to be established [3], the influence of chemical environment on its strength is not completely understood. In the present study we use topological indexes and an interacting quantum atoms (IQA) energy decomposition scheme [4] to explain how primary and secondary interactions affect the strength of a halogen bond. For this purpose, we use a series of model complexes described within DFT applying a dispersion correction. IQA energy decomposition scheme allows to extract pairwise interatomic contributions from total intermolecular interaction energy between halogen-bonded complex moieties and to estimate the proportion between Coulomb and exchange contributions [5]. Besides, the Source Function [6] analysis complements the description of the impact of chemical surroundings on the formation of halogen bond.
[1] P. Auffinger, F.A. Hays, E. Westhof, S. Ho, Proc. Nat. Acad. Sci, 2004, 101, 16789 – 16794
[2] P. Metrangolo, F. Meyer, T. Pilati, G. Resnati, G. Terraneo, Angew. Chem. Int. Ed., 2008, 47, 6114 – 6127
[3] P. Politzer, K.E. Riley, F.A. Bulat, J.S. Murray, Comp. Theor. Chem., 2012, 998, 2–8
[4] M. A. Blanco, A. Martín Pendás, E. Francisco, J. Chem. Theory Comput., 2005, 1, 1096 – 1109
[5] O.A. Syzgantseva, V. Tognetti, L. Joubert, in preparation
[6] R.F.W. Bader, C.Gatti, Chem. Phys. Lett., 1998, 287, 233 – 238
Verònica Postils, Eduard Matito, Marc Garcia-Borràs, Miquel Solà and Josep M. Luis
Institut de Química Computacional i Catàlisi and Departament de Química, Campus de Montilivi s/n, University of Girona, Girona, 17071, Spain
Characterization and Identification of Electrides
The electrides[1-2] are intriguing chemical species with an electron not formally assigned to any atom. This situation is, however, completely different to that given in a metal where the electrons are delocalized between positively charged metal ions. The electron in an electride acts as a formal anion, which is bonded to positively charged species in the molecule. This particular feature of electrides prompts very particular chemical and physical properties: they are powerful reducing reagents, exhibit exalted electric linear and non-linear optical properties as well as a particular magnetic behavior.
In this work, we analyze the electronic structure and the identification of several electride structures by means of the Quantum Theory of Atoms in Molecules (QTAIM) and the Electron Localization Function (ELF) [3]. Our results show that these tools make possible the classification of candidate species as electrides or not. It was already proved that one could distinguish the electride behavior in insulating high-pressure forms of alkali metals from ELF analysis [4] but now we show that with QTAIM and ELF is possible to characterize the electride behavior in all sorts of molecules.
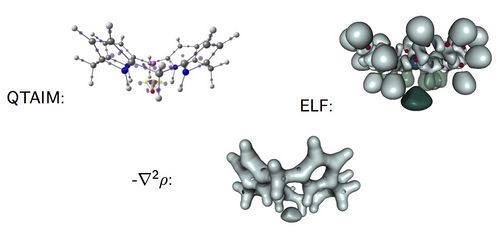
[1] Dye, J. L. Science, 2003, 301, 607-608.
[2] Dye, J. L. Science, 1990, 247, 663
[3] Matito, E.; Solà, M. Coord. Chem. Rev., 2009, 253, 647-665
[4] Marqués et al., Phys Rev. Lett. 2009, 103, 115501.
Mercedes Alonso, Paul Geerlings and Frank De Proft
Department of General Chemistry (ALGC), Free University of Brussels (VUB), Pleinlaan 2, Brussels, 1050, Belgium
Fine-tuning of the conformation of expanded porphyrins using conceptual DFT descriptors and non-covalent index
Density functional theory (DFT) is a powerful tool to examine a broad variety of structures and reactions involving increasingly larger molecules. Moreover, it is a well-suited theory for the introduction of chemical concepts, also known as reactivity indices [1]. Recently, we have shown that DFT calculations in combination with NCI analysis [2] are very useful in aiding the design of viable Möbius aromatic systems and molecular switches of expanded porphyrins [3-5]. These macrocycles can adopt a variety of intriguing structures, with Hückel, Möbius and twisted-Hückel topologies, which can be interconverted under certain conditions. The vast structural diversity exhibited by expanded porphyrins has led to diverse applications such as non-linear optical materials and molecular sensors, although the control of molecular topology is crucial for most of applications.
Conformational preferences and interconversion pathways in penta-, hexa- and heptaphyrins have been investigated using different hybrid functionals. The stability of Hückel and Möbius conformers depends on intramolecular hydrogen bonding, ring strain, aromaticity and steric effects of the meso-substituents. We proposed a set of descriptors to quantify independently the contribution of the different factors [3]. Two torsional parameters are used to quantify ring strain. Aromaticity has been quantified using energetic, structural, magnetic and reactivity criteria. Importantly, the relative hardness (a conceptual DFT descriptor) is found to be a good descriptor of the macrocyclic aromaticity in Hückel and Möbius porphyrinoids, whereas the dual descriptor is highly useful in predicting reactivity. Steric effects of the substituents are analysed in terms of the non-covalent interaction index (NCI). NCI is capable of distinguishing intramolecular hydrogen bonds, π- π interactions, CH···π and repulsive steric clashes, based on the peaks that appear in the reduced density gradient at low densities. While the relative stabilities of [4n + 2] expanded porphyrins are mainly governed by ring strain, intramolecular hydrogen-bonding and steric effects controls the stability of [4n] congeners. Appealingly, conformational control of pentaphyrins can be achieved by changing the type and number of meso-substituents [5].
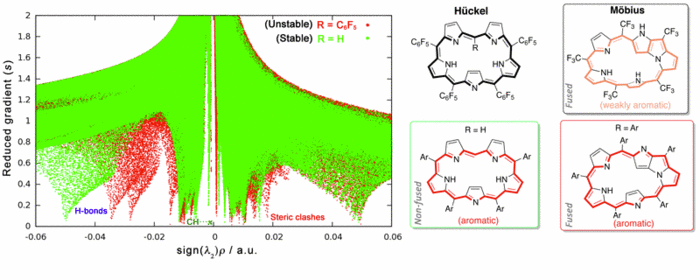
References
[1] P. Geerlings and F. De Proft, and W. Langenaeker, Chem. Rev. 103, 1793 (2003).
[2] E. R. Johnson, S. Keinan, P. Mori-Sánchez, J. Contreras-García, A. J. Cohen and W. Yang J. Am. Chem. Soc. 132 6498-6506 (2010).
[3] M. Alonso, P. Geerlings, and F. De Proft, Chem. Eur. J. 18 10916-10928 (2012).
[4] M. Alonso, P. Geerlings, and F. De Proft, Chem. Eur. J. 19 1617-1628 (2013).
[5] M. Alonso, P. Geerlings, and F. De Proft, J. Org. Chem. accepted. DOI: 10.1021/jo4003823.
Hélène GERARD
Université Pierre et Marie Curie - Paris 06
Interactions within heterobimetallic complexes
Main group metal cations (we will report about lithium, boron, aluminium, zinc and copper), when associated to anions (including carbanions) in non-aqueous solvent form a variety of structures going from separated ion pairs to fully polymerized arrangements. Theoretical exploration of the interactions at stake in these structures yields to a very contrasted picture depending not only of the system examined, but also of the analysis method at stake. Bridging the gap between these different models of molecular interactions brings essential information allowing to better understand the complexity of the solution behaviors.
Sílvia Simon, Laia Guillaumes
Institut de Química Computacional i Catàlisis (IQCC) and Departament de Química, Universitat de Girona, Campus de Montilivi, E-17071 Girona, Catalonia (Spain)
Delocalization Indices for Non-Covalent Interaction
Delocalization indices (DI) have been demonstrated to help of electronic structure of molecules, and more concretely in the analysis of HB nature.[1,2] Previous results pointed out that an electron-pair analysis in the framework of the AIM theory could be used to characterize hydrogen bonding in terms of the number of electrons shared between the atoms involved in the HB. As these indices are related to the polarity of the bond, they can be used to classify hydrogen bonds from weak to strong ones. Not only HB but also non-conventional hydrogen bond, eg. Dihydrogen bonded complexes, has been characterized by using DI.[3] Furthermore, these indices were also used in the study of Resonance Assisted Intramolecular Hydrogen Bond (RAHB). In that case, for a series of o-hydroxyaryl ketones, an intramolecular HB was formed giving an extra ring formation, finding out a good relation between DIs (between proton and proton acceptor atoms), and the stabilization energy when forming the HB.[4-6]
Different partition schemes can be defined to identify the atomic contributions to one property like DI. One of the partitions that has been gained more interest is to decompose the 3D space into atomic domains. Two main groups can be distinguished within this scheme, those with sharp boundaries between atomic domains (e.g. AIM, Voronio Cells, ELF) and those which assign a non-negative atomic weight functions to define the atomic basin in all the 3D space. The comparison of these 3D schemes will be also one of the points to discuss in the present work.
(1) X. Fradera, J. Poater, S. Simon, M. Duran, M. Sola, Theoretical Chemistry Accounts 108 (2002) 214.
(2) J. Poater, X. Fradera, M. Sola, M. Duran, S. Simon, Chemical Physics Letters 369 (2003) 248.
(3) D. Hugas, L. Guillaumes, M. Duran, S. Simon, Computational and Theoretical Chemistry 998 (2012) 113.
(4) M Palusiak, S. Simon, M. Sola, Journal of Organic Chemistry 71 (2006) 5241.
(5) M. Palusiak, S. Simon, M. Sola, Journal of Organic Chemistry 74 (2009) 2059.
(6) M. Palusiak, S. Simon, M. Sola, Chemical Physics 342 (2007) 43.
Arturo Espinosa
Departamento de Química Orgánica, Facultad de Química, Campus de Espinardo, Universidad de Murcia, 30100 Murcia, SPAIN.
Intra- and intermolecular NCIs balance in biscarbazole-trisurea (BC3U) receptors and their pyrophosphate complexes
As a part of recent studies on selective (optical, fluorescent or electrochemical) sensing of ions or neutral molecules, a number of derivatives of the novel biscarbazole-trisurea (BC3U) family of receptors have been prepared [1]. The prototypical peripherycally bis-p-nitrophenyl substituted term (Figure 1, left) shows a deeply coiled conformation in DMSO solution, according to the most stable computed [2] geometry obtained with the ORCA electronic structure package [3]. Addition of the biologically highly significant pyrophosphate anion selectively promotes remarkable experimental changes (optical, NMR shifts) that agree with the proposed computed (same level) structure (Figure 1, right). Complex formation requires a favourable energy balance between cleavage of several intramolecular NCIs in the receptor, involving both H-bonding and aromatic stacking (of either π,π- and T-types) and formation of new intermolecular (supramolecular) NCIs of mainly H-bonding type. All NCIs are displayed by means of colour-coded RDG (reduced density gradient) isosurfaces generated with the NCIplot program [4] and conveniently quantified by several typical bond-strength parameters.
Figure 1: Example of computed structures of a BC3U receptor and its pyrophosphate complex.
References
[1] G. Sánchez, A. Espinosa, D. Curiel, A. Tárraga, P. Molina, manuscript in preparation.
[2] COSMO(DMSO)/B3LYP-D3/def2-TZVP level of theory.
[3] ORCA An ab initio, DFT and semiempirical SCF-MO package. Written by F. Neese, Max Planck Institute for Bioinorganic Chemistry, D-45470 Mülheim/Ruhr, 2012. Version 2.9.1. Website: http://www.mpibac.mpg.de/bac/logins/neese/description.php. F. Neese, "The ORCA program system", WIREs Comput Mol Sci 2012, 2, 73–78.
[4] J. Contreras-García, E. R. Johnson, S. Keinan, R. Chaudret, J-P. Piquemal, D. N. Beratan, W. Yang. J. Chem. Theory Comput. 2011, 7, 625-632.
Rodrigo J. Alvarez-Mendeza**, Jorge M. del Campob, José L. Gázquezc and Alberto Velaa
a Departamento de Química, Cinvestav, Av. IPN 2508, Colonia San Pedro Zacatenco 07360, México, D.F. 07360 México b Departamento de Física y Química Teórica, Facultad de Química, Universidad Nacional Autónoma de México, México, D. F. 04510 México c Departamento de Química, Universidad Autónoma Metropolitana-Iztapalapa, Av. San Rafael Atlixco 186, México, D. F. 09340 México
Chemical interactions and dimensionless scalar fields
An analysis capable of identifying both strong and weak chemical interactions is presented. It is based on a generalization of the analysis presented by Zupan et. al (1,2) that consist in evaluating the integral
where is the diagonal element of the k-nt reduced density matrix and
is the Heaviside function.
represents the numbers of electrons in k-nt arrangement localized in the region constrained by the
scalar field isosurface
. For k=1, the derivative with respect to the φ yields the correspondent density of states and, at the same time, its bounds allow to localize the values that are chemically relevant for the scalar field.
Many examples to ilustrate the behavior of this analysis are presented using the exchange and correlation dimensionless gradients and the normalized electron localization function (3).
References
[1] A. Zupan, J.P. Perdew, K. Burke and M. Causa. Int. J. Quantum Chem. 61, 835 (1997)
[2] A. Zupan, K. Burke, M. Ernzerhof y J.P. Perdew, J. Chem. Phys. 106, 10184 (1997)
[3] Analysis of the generalized gradient approximation for the exchange energy. J. L. Gázquez , J. M. del Campo, S. B. Trickey, R. J. Alvarez-Mendez, A.Vela. Chapter in "Concepts and Methods in Modern Theoretical Chemistry, Vol. 1: electronic structure an reactivity". In honor of Professor B.M. Deb; S.K. Ghosh and P.K. Chattaraj eds. Taylor & Francis / CRC Press. (2013)
- Presenting autor
Rodrigo A. Cormanich,a,b Luke Crawford,a Michael Bühl,a Cláudio F. Tormenab and Roberto Rittnerb
Conformational analysis study of Ac-gly-NHMe, CF3-C(O)-gly-NHMe and Ac-gly-NH(Me)2 through theoretical calculations and NMR spectroscopy
a EastChem School of Chemistry, University of St Andrews, UK
b Chemistry Institute, University of Campinas, Brazil.
- email: rac22@st-andrews.ac.uk
In any attempt to understand protein folding processes, the characterization of the conformational preferences of short peptides constitutes an unavoidable first step. Indeed, there is a great interest in recognizing short peptide equilibrium geometries and to extrapolate the results obtained in this way to polypeptides and proteins [1]. However, free amino acid compounds and short peptide models (Ac-R-NHMe; R=amino acid) conformational geometry preferences have been commonly attributed to intramolecular hydrogen bonding (IHB) formation [2]. In particular, Ac-gly-NHMe has been the target of several studies [3], but despite steric and hyperconjugative effects, which are ubiquitous and influence the energies and geometries of even simpler systems [4], C5 and C7 Ac-gly-NHMe conformers stabilities have been explained solely by the possibility of a five- and a seven-membered N-H...O IHB formation, respectively. In this way, the present study aims to investigate the interactions that rule the conformational isomerism of Ac-gly-NHMe, CF3-C(O)-gly-NHMe and Ac-gly-N(Me)2 compounds through experimental (obtainment of 3JHH and 1JNH spin-spin coupling constants values) and theoretical calculations, using DFT funcionals with dispersion corrections (DFT-D and DFT-D3) and in the framework of the quantum theory of atoms in molecules (QTAIM) and natural bond orbitals (NBO). The results obtained by these methods indicate that Ac-gly-NHMe C5 conformer should not form an IHB, while conformer C7 forms a strong and stable N-H...O seven-membered IHB (Scheme 1). Indeed, Ac-gly-NHMe conformational preferences are ruled by IHB, but the interplay of hyperconjugative and steric interactions with IHB show to be important as well. Also, the CF3-C(O)-gly-NHMe C7 conformer has a weaker IHB force in comparison with the Ac-gly-NHMe C7 conformer, which increases the C5 conformer population and indicate that C7 stability is strongly seven-membered IHB force dependent. In addition, the Ac-gly-N(Me)2 compound cannot form a seven-membered N-H...O IHB and only the C5 conformer is observed for this compound. Thus, our analysis indicates that Ac-gly-NHMe conformational preferences are ruled by IHB in the gas phase, but that steric and hyperconjugative interactions have also an important contribution and cannot be ignored. QM/MM calculations are currently being performed in order to understand the behavior of these compounds in solution.
Ac-gly-N(Me)2 | |||||
C5 | C7 | C5 | C7 | C5 | |
![]() |
![]() |
![]() |
![]() |
![]() | |
%P | 12% | 88% | 44% | 55% | 100% |
Scheme 1: C5 and C7 Ac-gly-NHMe, CF3-C(O)-gly-NHMe and Ac-gly-N(Me)2 conformers molecular graphs obtained from the QTAIM calculations. Conformational populations (%P) and the eletronic density (ρ) values at the intramolecular hydrogen bonding BCP are indicated.
[1] M. F. Masman, R. F. Murphy, R. D., Enriz, A. M. Rodriguez J. Phys. Chem. 126 10682 (2007).
[2] R. A. Cormanich, L. C. Ducati, R. Rittner J. Mol. Struct. 1014, 12 (2012).
[3] H. Fujitani; A. Matsuura; S. Sakai; H. Sato; Y. Tanida J. Chem. Theory Comput. 5 1155–1165 (2009).
[4] R. A. Cormanich, Freitas M. P., J. Org. Chem.74, 8384 (2009).
Kasper Mackeprang
Department of Chemistry, University of Copenhagen
Weak intramolecular OH···π hydrogen bonding in methallyl and allyl carbinol
The weak intramolecular hydrogen bond in methallyl carbinol and allyl carbinol has been identified by intracavity laser photoacoustic spectroscopy as an observed redshift of the third OH-stretching overtone transition of the most abundant conformer of each molecule. Furthermore, the vibrational overtone spectrum indicates that the intramolecular hydrogen bond in methallyl carbinol is stronger than that in allyl carbinol as the redshift corresponding to the former molecule is larger. We have used the non-covalent interaction index to characterize and determine the strength of the intramolecular hydrogen bond in both molecules. It was confirmed that the strength of the hydrogen bond in methallyl carbinol was slightly larger than that in allyl carbinol.
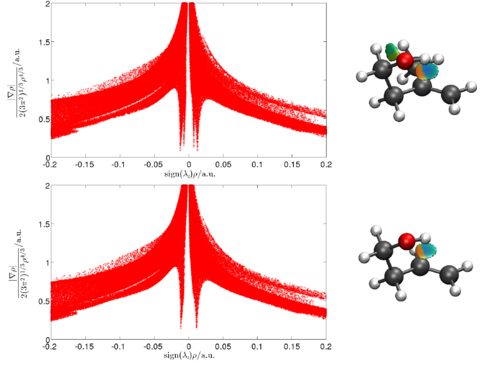
Marc Garcia-Borràs,a Sílvia Osuna,a Marcel Swart,a,b Josep M. Luis,a and Miquel Solàa
b Institució Catalana de Recerca i Estudis Avançats (ICREA), Pg. Lluís Companys 23, 08010 Barcelona, Spain
e-mail: marc.garcia@udg.edu
Intermolecular Dispersion Interaction Effects on the Diels-Alder Endohedral Metallofullerene Monoadduct stabilities. The Cp/Cp*-La@C2v-C82 example.
In the present work, we have studied the regioselectivity of the Diels-Alder (DA) addition of cyclopentadiene (Cp) and 1,2,3,4,5-pentamethylcyclopentadiene (Cp*) on La@C2v-C82 EMF at the B3LYP-(D)/TZP//BLYP-D/DZP level of theory.[4] Experimentally, the major product of the Cp* addition was characterized by X-ray crystallography.[5] By contrast, the product of the Cp DA reaction was still not known.[6] Moreover, both products present very different experimental stabilities being the Cp* adduct thermally much more stable than the Cp one, which rapidly decomposes into reactants at room conditions.[5] This different behavior suggests that the two products differ from each other.
Our results show that, surprisingly, the regioselectivity of both reactions mentioned above is equivalent, the same C-C bond of the EMF being the most favored attacked during the DA reaction. And, moreover, their thermal stability is practically the same with similar retro-reaction barriers. The different product stability is caused by the formation of the initial reactant complex due to the intermolecular dispersion interactions. In the Cp* case, in contrast to the Cp case, the formation of the adduct is largely favored,, being the intermolecular dispersion energy term much larger for the Cp*-EMF adduct than for the Cp-EMF system. This implies a rapid dissociation of the latter, and on the contrary a dynamic equilibrium between the initial complex and the final product for the Cp* diene. Thus, we have found that the key role played by the initial reactant complex finally determines the thermal stability of the final adducts in this particular cycloaddition reaction.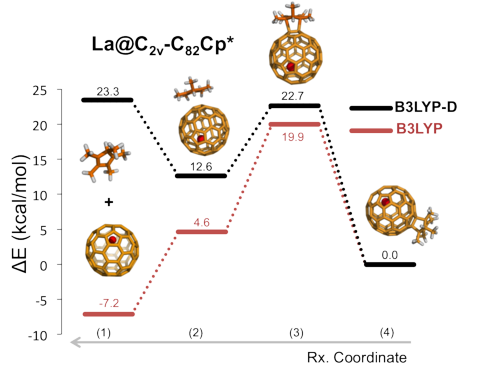
[1] S. Osuna, M. Swart, M. Solà, Phys. Chem. Chem. Phys. 2011, 13, 3561.
[2] M. Garcia-Borràs, S. Osuna, J. M. Luis, M. Swart, M. Solà, Chem. Eur. J. 2012, 18, 7141.
[3] M. Garcia-Borràs, A. Romero-Rivera, S. Osuna, J. M. Luis, M. Swart, M. Solà, S. J. Chem. Theory Comput. 2012, 8, 1671.
[4] M. Garcia-Borràs, M. Swart, J. M. Luis, M. Solà, Chem. Eur. J. 2013, 19, 4468.
[5] Y. Maeda, S. Sato, K. Inada, H. Nikawa, M. Yamada, N. Mizorogi, T. Hasegawa, T. Tsuchiya, T. Akasaka, T. Kato, Z. Slanina, S. Nagase, Chem. Eur. J. 2010, 16, 2193-2197.
[6] Y. Maeda, J. Miyashita, T. Hasegawa, T. Wakahara, T. Tsuchiya, T. Nakahodo, T. Akasaka, N. Mizorogi, K. Kobayashi, S. Nagase, T. Kato, N. Ban, H. Nakajima, Y. Watanabe, J. Am. Chem. Soc. 2005, 127, 12190-12191.
Eduard Matito, Ferran Feixas, Juan M. Barroso, Miquel Solà and Jesus M. Ugalde
Institut de Química Computacional i Catàlisi and Departament de Química, Universitat de Girona, Campus de Montilivi s/n, 17071 Girona, Catalonia, Spain
Multicenter Bond Index: a versatile tool to characterize electron delocalization and aromaticity.
Multicenter indices (MCI) [1-10] are used in a number of situations such as the analysis of conjugation and hyperconjugation effects [7], to identify agostic bonds, to account for electron distributions in molecules [4-5] or to study aromaticity in both organic [9] and all-metal compounds [10-11]. The formula of MCI involves the intuitive n-order central moment of the electron population [12-13], which depends on the n-order RDM. The calculation of the n-RDM is overwhelmingly expensive for correlated wavefunctions and, therefore, there are so few studies of MCI available in the literature. In this talk we review the applications of MCI and suggest a new approximation for the 3-RDM that provides more accurate MCI values than the approximations suggested thus far in the literature [15-16].
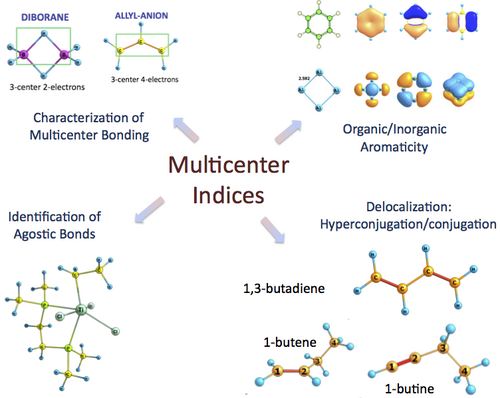
[1] Giambiagi, M. et al. A. P. Phys. Chem. Chem. Phys. 2 (2000) 3381.
[2] Bultinck, P et al. J. Phys. Org. Chem. 18 (2005) 706.
[3] Cioslowski, J. et al. J. Phys. Chem. A 111 (2007) 6521.
[4] Pendás, A. M. et al. Phys. Chem. Chem. Phys. 9 (2007) 1087.
[5] Pendás, A. M. et al. J. Chem. Phys. 127 (2007) 144103.
[6] Ponec, R. et al. J. Phys. Chem. A 101 (1997) 1738.
[7] Feixas, F. et al. J. Phys. Chem. A 115 (2011) 13104.
[8] Bultinck, P. Faraday Discuss. 135 (2007) 347.
[9] Feixas, F. et al. J. Comput. Chem. 29 (2008) 1543.
[10] Feixas, F. et al. Theor. Chem. Acc. 128 (2011) 419.
[11] Feixas, F. et al. J. Chem. Theory Comput. 6 (2010) 1118.
[12] Giambiagi, M. et al. Struct. Chem. 1 (1990) 423.
[13] Francisco, E. et al. J. Chem. Phys 126 (2007) 094102.
[14] Feixas, F. et al. in preparation.
[15] Colmenero, F. et al. Int. J. Quantum Chem. 51 (1994) 369.
[16] Mazziotti, D. A. Phys. Rev. A 57 (1998) 4219.
Alberto Otero-de-la-Roza and Erin Johnson
Chemistry and Chemical Biology, School of Natural Sciences, University of California, Merced, 5200 North Lake Road, Merced, California 95343, USA
Exchange and correlation effects on non-covalent interactions
In the last decade, great progress has been made towards accurate representation of non-covalent interactions using density-functional theory (DFT). Different exchange-correlation energy approximations yield widely disparate pictures of intermolecular interactions, but can be successfully corrected by addition of a damped dispersion energy term, as obtained with the exchange-hole dipole moment model (XDM)[1,2,3,4]. However, there are several open questions regarding this procedure: What is the rationale behind some exchange-correlation functionals working better than others when paired with the dispersion correction? What is the source of these discrepancies in real space? How does this error scale with increasing system size? Are many-body interactions correctly treated at the DFT level? Is it necessary to include a three-body dispersion term[3]? In this talk, I will present our recent work addressing these challenges.
[1] A. D. Becke and E. R. Johnson, J. Chem. Phys. 122, 154104 (2005)
[2] A. D. Becke and E. R. Johnson, J. Chem. Phys. 127, 154108 (2007)
[3] A. Otero-de-la Roza and E. R. Johnson, J. Chem. Phys. 138, 054103 (2013)
[4] A. Otero-de-la Roza and E. R. Johnson, J. Chem. Phys. (submitted)
Christine Lepetit,aJordi Poater,b Miquel Solà,bJulia Contreras-Garcia,cYves Canac,aDavit Zargarian,d Remi Chauvin a
a Laboratoire de Chimie de Coordination du CNRS, BP 44099, 205 route de Narbonne, 31077 Toulouse Cedex 4. France b Institut de Quimica Computacional and Departament de Quimica, Universitat de Girona, Campus de Montilivi, 17071 Girona, Catalonia, Spain c Laboratoire de Chimie Théorique, (UPMC) CC137, 4, place Jussieu 75252 Paris cedex 05. France dDépartement de Chimie, Université de Montréal, Québec H3C 3J7. Canada
- christine.lepetit@lcc-toulouse.fr
Theoretical studies of the metal-phenylene interaction in a P(CH)P pincer rhodium(I) complex
Amidiniophosphines are cationic electron-poor ligands in transition metal complexes with potential original catalytic properties [1]. In the pincer ligand series, based on the meta-phenylene bridge, the first unsymmetrical nickel(II) complex with a phosphinite and an imidazoliophosphine extremities was recently reported [2]. However switching to rhodium(I) and to two cationic imidazoliophosphine extremities, a P(CH)P pincer complex 2 was obtained instead of the targeted PCP pincer complex 1 [3]. On the basis of DFT studies, an alternative pathway to the usually invoked C-H oxidative addition, is proposed to explain the formation of 2 and its reluctance to yield the PCP pincer 1 [3-4].
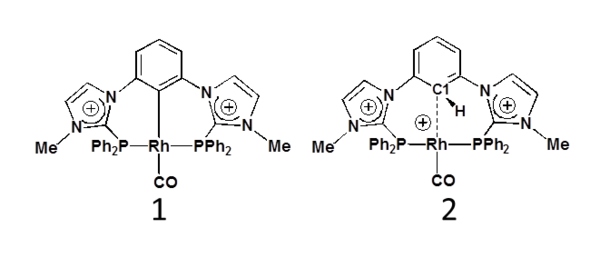
Experimental and calculated spectra of 2 are in good agreement with a weak rhodium-phenylene interaction. The signature of a Rh-h1-C1 bonding is found from ELF (electron localization function) topological analysis. Further comparative studies using molecular orbital analysis and other theoretical tools such as NCI (non covalent interactions) analysis and MCI (multicenter delocalization indices) [5] allow for refining the characterization of this particular metal-aryl bonding. To the best of our knowledge, it has been little discussed in the literature up to now, although it may be inserted as a missing entry in the well-known series of agostic and anagostic interactions. [6]
References
[1] Y. Canac, C. Maaliki, I. Abdellah, R. Chauvin New. J. Chem. 2012, 36, 17.
[2] B. Vabre, Y. Canac, C. Duhayon, R. Chauvin, D. Zargarian Chem. Commun. 2012, 48, 10446.
[3] C. Barthes, C. Lepetit, Y. Canac, C. Duhayon, D. Zargarian, R. Chauvin Inorg. Chem. 2013, 52, 48.
[4] B. Vabre, M. L. Lambert, A. Petit, D. H. Ess, D. Zargarian Organometallics 2012, 31, 6041.
[5] a) P. Bultinck, R. Ponec, S. Van Damme J. Phys. Org. Chem. 2005, 18, 706. b) I. Mayer, P. Salvador Chem. Phys. Lett. 2004, 383, 368.
[6] M. Brookhart, M. L. H. Green, G. Parkin PNAS, 2007, 104, 6908.
Piotr de Silva1,2, Jacek Korchowiec1, Tomasz A. Wesolowski2
1K. Gumiński Department of Theoretical Chemistry, Faculty of Chemistry, Kraków Jagiellonian University, R. Ingardena 3, 30-060 Kraków, Poland 2Université de Genève, Département de Chimie Physique 30, quai Ernest-Ansermet, CH-1211 Genève 4, Switzerland
Probing Aromaticity and Non-Covalent Interactions with Single Exponential Decay Detector (SEDD)
Single Exponential Decay Detector (SEDD) [1] is an orbital-free descriptor of bonding patterns in molecular systems. Calculation of SEDD requires only the density ans its first and second derivatives

SEDD is able to distinguish such localized elements of electronic structure like bonds, lone pairs and core electrons. In this contribution the recent applications of SEDD are outlined. This includes investigating electron delocalization in aromatic system [2] as well as non-covalent interactions.
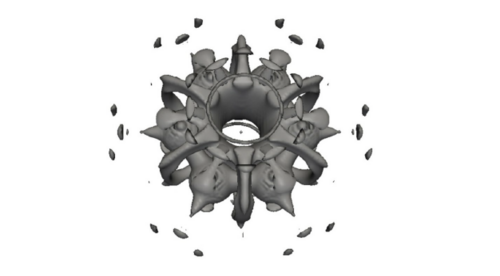
[1] P. de Silva, J. Korchowiec, T. A. Wesolowski, ChemPhysChem 2012, 13, 3462-3465.
[2] P. de Silva, J. Korchowiec, N. Ram J.S., T. A.Wesolowski, Chimia (in press).
Ferran Feixas, Steffan Lindert, Meekyum Kim, William Sinko, J. Andrew McCammon
Department of Pharmacology, University of California, San Diego, 9500 Gilman Drive, La Jolla, 92039, California, USA'
The role of cis-prenyl diphosphate synthases in bacterial cell-wall biosynthesis: non-covalent interactions and its implications for drug discovery
The rise in resistance to antibiotics is becoming a major problem. Thus, there is a need for new drugs, with new chemical structures, that target alternative enzymes. In this work, we focus on the cis-farnesyl-diphosphate synthase (cis-FPPS) and cis-decaprenyl-diphosphate synthase (cis-DPPS), two enzymes found in Mycobacterium Tuberculosis that work in series to synthesize decaprenyl-diphosphate, which is essential for the bacterial cell-wall biosynthesis. Interestingly, both enzymes present similar 3D-structures and catalytic mechanism. However, the length of the products synthesized by the two enzymes is remarkably different, cis-FPPS produces a short 15-carbon product called farnesyl-diphosphate, while cis-DPPS generates a larger 50-carbon decaprenyl-diphosphate.
The first aim of this work is to perform MD simulations and docking studies to analyze the influence of protein flexibility on the design of cis-prenyl-diphosphate synthase inhibitors. To this end, we make use of the Relaxed Complex Scheme (RCS), a type of ensemble docking, which relies on previously determined conformations from MD simulations to perform docking studies to select the most promising compounds that will be tested experimentally.[1] This strategy has been successfully applied to design promising inhibitors in related systems such as undecaprenyl diphosphate synthases (UPPS). [2] The second aim is to study in deep detail the interaction between the receptor and some of the experimentally active inhibitors obtained in the first step. In particular, we focus our attention on the analysis of the non-covalent interactions on the hydrophobic cavity of the active site of cis-FPPS and DPPS enzymes. We will see how a deeper understanding of intermolecular drug-receptor non-covalent interactions can help us on the drug discovery process.
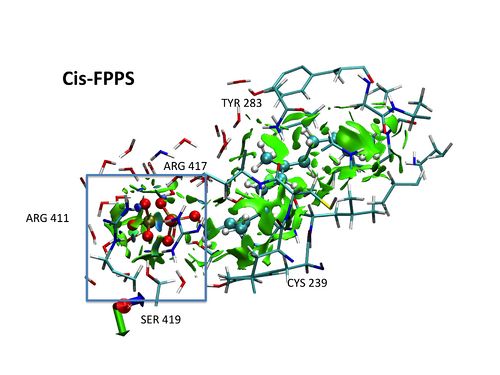
[1] W. Sinko, S. Lindert, J. A. McCammon, Chem. Bio. Drug. Des. 2013, 41.
[2] W. Zhu, Y. Zhang, W. Sinko, M. E. Hensler, J. Olson, K. J. Molohon, S. Lindert, R. Cao, K. Li, K. Wang, Y. Wang, Y. Liu, A. Sankovsky, C.A.F. Oliveira, D. A. Mitchell, V. Nizet, J.A. McCammon, E. Oldfield, PNAS, 2013, 123
Your name
Your institution
Your title
Your abstract
González-Veloso1, D. Josa1, J. Rodríguez-Otero1, E. M. Cabaleiro-Lago2
1Centro de investigación en Química Biolóxica e Materiais Moleculares, CIQUS, Universidade de Santiago de Compostela Rúa Jenaro de la Fuente s/n, Santiago de Compostela, 15782, SPAIN 2Departamento de Química Física, Universidade de Santiago de Compostela Facultade de Ciencias, Avda. de Alfonso X o Sabio s/n, Lugo, 27002, SPAIN
Theoretical study of the interaction between planar and curved compounds: perfluoro-ortho-phenylenmercury and corannulene
Recently, Filatov et al.[1] have performed an experimental study of the interaction of perfluoro-ortho-phenylenemercury [Hg3] with corannulene and monoindenocorannulene. The first compound is planar; however the other two are curved bowls. Therefore, these systems allow studying the mutual structural influences between surfaces of planar and nonplanar molecules upon their interaction. This kind of interactions has generated considerable interest in materials chemistry. According to the experimental study, significant geometry adjustments of both interacting partners were found in the solid-state complexes. So, Hg3 adopts highly bent configurations, which are substantially deviating from planarity, whereas flattening of the bowl-shaped polyarenes is rather more modest. corannulene Hg3
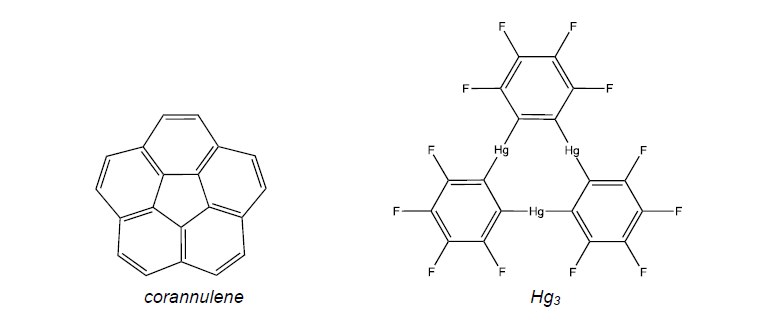
In the present work a theoretical study was performed at the DFT and ab initio levels to test the experimental fact, finding that corannulene flattened slightly while [Hg3] curved more sharply. It also checked that the union of these atoms is due basically to dispersion energy between molecules. For that reason the DFT functionals have been corrected by an empirical dispersion term as designed by Grimme (DFT-D).[2] Finally it was found that the substituents on the [Hg3] also affect the interaction energy between these monomers, as well as the deformation between the molecules.
[1] A. S. Filatov, E. A. Jackson, L. T. Scott, M. A. Petrukhina, Angew. Chem. Int. Ed. 2009, 48, 8473.
[2] S. Grimme, S. Ehrlich, L. Goerik, J. Comput. Chem., 2011, 32, 1456.
Roberto Álvarez Boto1, Mónica Calatayud2, Julia Contreras García2 and A. Martín Pendás1
1Universidad de Oviedo. Spain
2Laboratoire de Chimie Théorique, Université Pierre et Marie Curie and CNRS, Paris, France
The role of packing in the platinum-octylamine system: a NCI analysis
The binding of long alkyl molecules to metal surfaces has been widely investigated due to its many technological applications such as films, electronic junctions, coating or tribology[1]. The intermolecular interactions play a key role not only in the physicochemical properties of these systems, but also in their stabilization. These interactions come from the vicinity of the neighboring molecules forming a two-dimensional layer. Despite the amount of investigations concernig metal-supported self assembled monolayers (SAMs), little is known about the dispersion forces present in these 2D arrangements. In the present work the platinum (100)- octylamine(NH2C8H17)has been chosen as model system to show the role of packing. A bonding analysis based on NCI index [2] was applied to both, the octylamanine dimer and the 2D arrangement to ilustrate the nature of the intermolecular interactions. Additionally, a detailed study on the structural parameters of the alkyl-system is reported to gain understanding the factors affecting the stability of the system. All the calculations were carried out at DFT theory level combined with Grimme D2 approach.
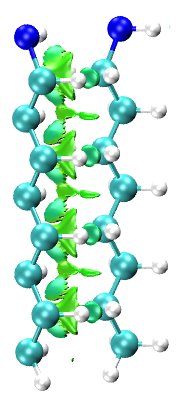
[1] Caroline Salzemann; Christophe Petit. Influence of Hydrogen on the Morphology of Platinium and Palladium Nanocrystals. Langmuir, 2012, 28, 4835-4841.
[2] E. R. Johnson, S. Keinan, P. Mori-Sánchez, J. Contreras-García, A. J. Cohen and W. Yang J. Am. Chem. Soc. 132, 6498-6506 (2010).
Robin Chaudret, Natacha Gillet, Pan Wu, Julia Contreras-Garcia, Jean-Philip Piquemal, Weitao Yang
IFP Energies nouvelles 1 et 4 Av de Bois-Préau 92852 Rueil-Malmaison CEDEX
Non covalent interaction within reactive and dynamic systems : a presentation of ELF/NCI and aNCI
Weak interactions play a predominant role in various problems such as ligand docking, catalytic stereoselectivity or formation of supramolecular structures but also in initiating chemical reactions. These interactions can be very different in nature or energy but they all share a same characteristic: the difficulty for a chemist to localize them. Indeed their energies are really small and therefore their geometries or any other experimental properties are subject to strong variations. It is then particularly difficult to characterize them energetically or spatially. For the theoretical chemist, the only way to identify them is to use very precise (and computationally expensive) methods which can be incompatible with the size of a realistic systems, the exploration of the different possible conformations or the research of a reaction profile. The recently developed NonCovalent Interaction (NCI) index[1-3] allows a characterization and an estimation of these weak interactions. This approach is based on the study of the reduced density gradient as a function of the density and allows a visualization of the non-covalent interactions. The latter are colored in function of their strength. The first part of the talk will briefly describe the NCI analysis. This new methods appear therefore perfectly adapted to visualize the early steps of a reaction (when the interaction is still quite weak) but when the interaction gets stronger, the NCI validity decreases. In such a range, however, other approaches such as the Electron Localization Function[4-6] (ELF) have shown great success. The coupling of the two methods should allow an understanding of every steps of the reaction, from the weak interaction up to the bond formation. The second part of the talk will describe the ELF-NCI[7] approach and demonstrate its interest on several reaction mechanisms.
Finally, the importance of the environment effect on a reaction is now largely recognized and is mainly studied through QM/MM calculations. If NCI can localize the weak interactions, it is, however, strongly dependent on the input structure, which makes it unsuitable for analyzing fluctuating systems such as solute/solvent interactions or protein/ligand binding. In the last part of the talk we will present the new average Non Covalent Interaction (aNCI) approach[8] especially designed to treat such problems. The theory and several examples including benzene solvation, solvent effect along a reaction mechanism and ligand docking will be presented.
[1] Johnson, E. R.; Keinan, S.; Journal of the American Chemical Society 2010, 132, 6498-6506.
[2] Contreras-Garcia, J.; Johnson, E. R.; Journal of Chemical Theory and Computation 2011.
[3] Contreras-GarcÃa, J.; Yang, W.; Johnson, E. R. The Journal of Physical Chemistry A 2011, 115, 12983-12990.
[4] Becke, A. D.; Edgecombe, K. E. Journal of Chemical Physics 1990, 92, 5397-5403.
[5] Silvi, B.; Savin, A. Nature 1994, 371, 683-686.
[6] Chaudret, R.; Cisneros, G. A.; Chemistry-A European journal 2011, 17, 2833-2837.
[7] Gillet, N., Chaudret, R.; J. Chem. Theory Comput., 2012, 8 3993–3997.
[8] Wu, P., Chaudret, R.; J. Chem. Theory Comput., Article ASAP; DOI: 10.1021/ct4001087.
Gabriele Saleh
Department of Chemistry, Università degli Studi di Milano, via C.Golgi 19, 20159 Milano Italy
exploring non-covalent interactions in molecular crystals through the analysis of their experimental electron density distribution
The so-called ‘NCI descriptor’ introduced by Johnson et al. [1], besides allowing an easy-to-catch pictorial visualization of Non-Covalent Interactions (NCI), presents the big advantage of being based on electron density (ED) distribution, allowing therefore to investigate NCI theoretically and experimentally on the same ground. Indeed, ED is an observable and measurable quantity (from X-ray diffraction experiments) which, at the same time, can be straightforwardly obtained from the wavefunction. Quite recently, we have applied the 'NCI descriptor' for the first time to experimentally derived ED [2]. We have also compared the experimental results with the one obtained from fully periodical DFT calculation, to assess the reliability of experimentally derived NCI picture. Moreover, we have contrasted the picture obtained from 'NCI descriptor' with the one obtained from Quantum Theory of Atoms in Molecules [3] to show how the two approaches may fruitfully complement each other in providing additional insight into the nature of a given NCI. In a separated work [4] we carried out a systematic comparison between the NCI picture obtained from promolecular ED (i.e. the ED obtained by superposing the atomic densities) and the one from experimentally derived ED, to explore to what extent the promolecular ED can be used to investigate NCI, as proposed by Contreras et al. [5] . The results relative to the application of NCI descriptor to experimental, theoretical and promolecular ED of several system will be presented. In addition, preliminary results related to a variation of the classical 'NCI descriptor', exploiting the energy density, will be shown.
References: [1]E. R. Johnson, S. Keinan, P. Mori-Sanchez, J. Contreras-Garcia, A. J.Cohen, W. Yang, J. Am. Chem. Soc. 2010, 132, 6498 – 6506 [2]G. Saleh, C. Gatti, L. Lo Presti, J. Contreras-Garcia, Chem. Eur. J. 2012, 18, 15523–15536 [3]R. F. W. Bader, Atoms In Molecules: A Quantum Theory, Clarendon Press, Oxford, 1990 [4]G. Saleh, C. Gatti, L. LoPresti, Comput. Theor. Chem. 2012,998,148–163 [5]J. Contreras-Garcia, E.R. Johnson,S. Keinan, R.Chaudret , J-P. Piquemal, D.N. Beratan, W. Yang, J. Chemical Theory and Computation, 2011, 7, 625-632
Your name
Your institution
Your title
Your abstract
Your name
Your institution
Your title
Your abstract